April 1961 Radio-Electronics
[Table of Contents]
Wax nostalgic about and learn from the history of early electronics.
See articles from Radio-Electronics,
published 1930-1988. All copyrights hereby acknowledged.
|
As mentioned in this 1961
Radio-Electronics magazine article, fuel cells have been around for a
long time. Francis T. Bacon (not the 17th century philosopher
Francis Bacon) built his first fuel cell
sometime in the 1940s. He designed the fuel cells for the Apollo 11 and other
spacecraft. President John Kennedy's famous
moon speech
was delivered a month after this article, and predated the
moon landing by
more than eight years. Most critical technology experiences an evolutionary
period spanning decades from inception to practical application. That time is
being reduced with the advent of computers and larger numbers of people working
on the problems. Just as fuel cells have become ubiquitous in electrical power
generation processes, so, too, will other technologies that were once considered
to be pie-in-the-sky bits of science fiction - like nuclear fusion reactors (aka
tokamaks). Researchers are now very near the breakeven point necessary to enable
self-sustaining, controlled reactions.
Fuel Cells - Tomorrow's Electric Generators?
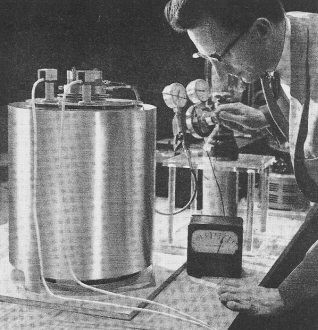
An electric battery with a gas tank
By Leonard G. Austin
A fuel cell operates on the same electrochemical principle as the ordinary flashlight
battery. However, a fuel is fed into the cell continually so it never runs down
as long as fuel and air are available. Unlike lead-acid or nickel-iron-alkaline
batteries, fuel cells do not have to be recharged electrically - "recharging" simply
consists of refilling the fuel tank. Three major fields are open to fuel cells.
They could be used as non-expendable cells providing cheaper and more reliable electricity
than conventional batteries. Used to power electric motors they could take the place
of gasoline and diesel engines for road and rail transport. Finally, large fuel-cell
installations might generate electric power with greater fuel efficiency and lower
cost than central power plants using boiler-steam turbine-generator systems,
Fuel cells can generate small or large amounts of electricity at efficiencies
up to 80%. In the past 5 years there has been an explosion of research on the cells.
All major companies in the automobile, aircraft, heavy chemical, battery, electrical
and oil industries are in keen competition.
The most highly developed fuel cells at present use hydrogen as fuel, oxygen
as oxidizer and concentrated potassium hydroxide as the electrolyte. This type of
cell will be used to explain the principle of operation of fuel cells. Fig. 1 shows
a simple cell of this type. The electrical energy we take from the cell as current
and voltage is supplied by the chemical energy of the combination of hydrogen and
oxygen to form water. Normally, hydrogen and oxygen will not react until they are
heated to over 500° C. The trick in combining hydrogen and oxygen at low temperature
to give electrical energy is to make the reaction proceed in a series of catalyzed
steps, in one of which an electron is transferred across a circuit. (The catalysis
involves a surface which holds reactants and allows them to react at much lower
temperatures.)
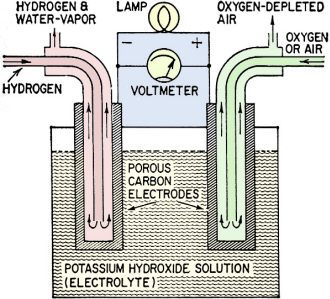
Fig. 1 - Typical hydrogen-oxygen fuel cell.
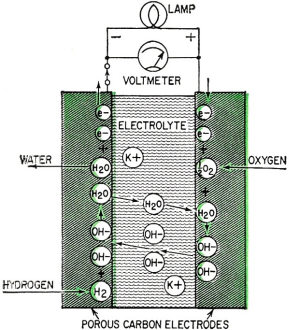
Fig. 2 - What happens in a fuel cell when the circuit is closed.
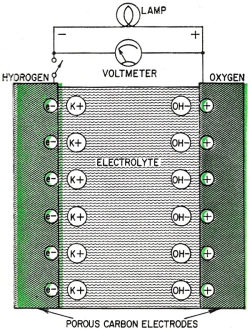
Fig. 3 - Open circuit stops reaction.
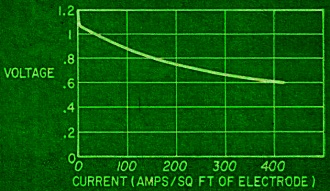
Fig. 4 - Current-voltage curve of a hydrogen-oxygen fuel cell.
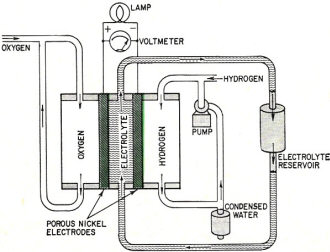
Fig. 5 - Francis T. Bacon developed this hydrogen-oxygen cell.
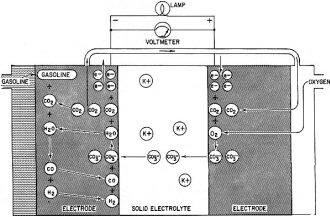
Fig. 6 - High-temperature fuel cell uses "solid" electrolyte of potassium carbonate
and operates at temperatures above 500°C.
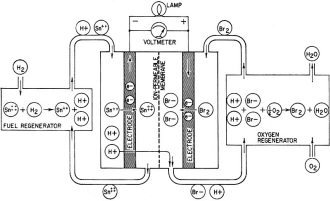
Fig. 7 - Redox type fuel cell.
Fig. 2 shows the series of steps in a fuel cell. It has two porous electrodes
separated by a potassium hydroxide electrolyte. On the negative side of the cell,
hydrogen gas diffuses through the electrode, and hydrogen molecules (H2),
assisted by a catalyst embedded in the electrode surface, are adsorbed on the surface
in the form of hydrogen atoms (H). The atoms react with hydroxyl ions (OH-)
in the electrolyte to form water, giving up electrons to the electrode in the process.
The water goes into the electrolyte. This reaction is also aided by the catalyst.
The flow of these electrons through the external circuit to the positive electrode
is the electric output of the cell and supports the oxygen half of the reaction.
On the positive side of the cell oxygen (O2) diffuses through the electrode
and is adsorbed on the electrode surface. In an indirect reaction, the adsorbed
oxygen, plus the incoming electrons and water in the electrolyte, form hydroxyl
ions. Here again a catalyst helps the reaction along. The hydroxyl ions complete
the circle by migrating through the electrolyte to the hydrogen electrode.
If the external circuit is open, the hydrogen electrode accumulates a surface
layer of negative charges that attracts a layer of positively charged sodium or
potassium ions in the electrolyte. An equivalent process at the oxygen electrode
similarly balances its accumulated positive charge (Fig. 3). These electrical "double
layers" prevent further reaction between the gases and the electrolyte. These layers
also provide the potential that forces the electrons through the external circuit
when an external connection is made.
Theoretically, a fuel cell can convert the available chemical energy of the fuel
to electrical energy at almost 100% efficiency. In practice, energy has to be used
to overcome the activation energy barriers of the reactions in the cell, overcome
the ohmic resistance of the electrolyte and supply the transport energy of feeding
the gases through the porous electrode. High current drain makes these losses greater
and the cell voltage decreases from the open circuit value (Fig. 4). Efficiencies
of 60% to 80% at working currents can be obtained. If we compare this efficiency
level with 25-30% for the normal gasoline engine and 35-40% for central electricity
power generation, we see why fuel cells are exciting so much interest.
Union Carbide has developed a hydrogen-oxygen cell which operates at about 60°
C. The electrodes consist of porous carbon impregnated with catalysts: fine particles
of platinum or palladium in the hydrogen electrode and cobalt oxide, platinum or
silver in the oxygen electrode. To prevent flooding the pores by the electrolyte
- which would cut down the active surface - the electrodes are waterproofed with
paraffin wax. The wax prevents the water from creeping into the pores. To bring
the electrodes closer together and thus speed ion transport, they are usually arranged
as concentric tubes or adjacent plates. These cells are being used by the Army as
portable, quiet power generators.
General Electric has developed a low-temperature hydrogen-oxygen cell which uses
an ion-exchange membrane instead of a liquid electrolyte. The membrane transports
a hydrogen ion (H+) to the oxygen electrode where it reacts to form water.
The electrodes are thin sheets of porous platinum-palladium. This cell is suitable
only for small current outputs because of the low ionic conductivity of the membrane;
however, it is thin and about 60 cells stacked in series are but 1 foot long.
If the electrochemical reactions are speeded up by running the cells at higher
temperatures, hydrogen-oxygen cells are capable of much greater electrical outputs
per unit volume. To prevent electrolyte boiling at high temperatures, the whole
cell must be pressurized. A cell of this type, developed by Francis T. Bacon of
Cambridge University (England), operates at about 250° C with gas pressures
that run as high as 800 pounds per square inch (Fig. 5). The porous nickel electrodes
are about 1/16 inch thick, usually in the form of disks or plates. The reaction
area is a thin, porous surface layer on the electrode. The electrolyte is a solution
of potassium hydroxide and could enter these pores but for the pressure differences
within the electrode which keep the electrolyte from flooding the larger pores in
the body of the electrode. This fuel cell produces six times as much power per cubic
foot as the low-temperature hydrogen-oxygen cells.
High-Temperature Cells
Although hydrogen-oxygen cells have been well developed, they have two big disadvantages.
Hydrogen is costly and bulky. Hydrogen stored at 150 atmospheres pressure contains
less than one-hundredth the energy of the same volume of gasoline at normal conditions.
Fuel cells must "burn" cheap fuels (natural gas, vaporized gasoline) to produce
economical power on a large scale. Extracting energy from such fuels at present
needs operating temperatures above 500°C. Since water-base electrolytes boil
away at these temperatures, the electrolyte usually consists of some molten salt,
sodium or potassium carbonate, mixed with lithium carbonate to lower the melting
point for example. In the most efficient high-temperature cells, the electrolyte
is held in a matrix of porous refractory material. The electrodes, made of a variety
of metals or metallic oxides, are tightly pressed against the "solid" electrolyte.
In these cells the fuel is probably "cracked" to hydrogen and carbon monoxide
by reaction with steam and carbon dioxide, which the fuel cell produces as byproducts.
This cracking may be done outside or inside the cell.
In the current-generating reaction, the hydrogen and carbon monoxide diffuse
into the cell at the negative electrode, react with carbonate ions in the electrolyte
and form carbon dioxide and water while giving up electrons to the electrode. At
the positive electrode, oxygen or air takes up the electrons flowing in from the
external circuit, reacts with the carbon dioxide and produces the carbonate ions.
Migration of carbonate ions through the electrolyte from the positive to the negative
electrode completes the circuit (Fig. 6).
High-temperature fuel cells, intensively investigated only since World War II,
still perform poorly. The best of them produce no more than half the yield of the
low-temperature hydrogen-oxygen cell and a twelfth the yield of the Bacon cell.
However, the progress already made in hydrogen-oxygen cells suggests that further
research can greatly improve the performance of high-temperature cells.
Another cell which might be able to use cheap fuels is the "redox" cell (named
for reduction and oxidation). In this cell the fuel and oxygen are made to react
with other substances in "regenerators" outside the cell to produce chemical intermediates,
which in turn generate current in the cell. The overall reaction is the same as
that of combustion, however, because the intermediates are regenerated. A typical
cell of this type was developed in England under the leadership of Sir Eric Rideal.
It uses tin salts and bromine as intermediates (Fig. 7). The fuel reduces (adds
electrons to) tin ions, which then give up the added electrons to the negative electrode
and return to react with more fuel. The oxygen similarly oxidizes (takes electrons
from) bromide ions, converting them to bromine, which then takes up electrons from
the positive electrode and returns as bromide ions for regeneration. A similar cell,
using titanium salts instead of tin, is under development by the General Electric
Co. The stumbling block with this type of cell is that most fuels cannot react quickly
enough in the regenerator to keep up the current flow. Investigations of catalysts
to speed up the reaction are being made by G-E and by the Pennsylvania State University.
Special-Purpose Cells
Several other types of cells and many fuels are being investigated. Hydrazine
(N2H2) and ammonia (NH3) are being used as fuels
in hydrogen-oxygen type cells. They act as convenient hydrogen carriers; a catalyst
in the electrode splits them into hydrogen and nitrogen. Methyl alcohol (wood spirit)
has been used in similar cells. Although alcohol is not cheap enough to use for
large-scale electricity production, it is certainly cheap and convenient enough
to replace expensive nonrechargeable dry cells. Allis-Chalmers Co. has developed
a cell which runs at 60°C on a fuel mixture of propane and hydrogen. It uses
potassium hydroxide electrolyte and activated nickel electrodes. A 20-horsepower
tractor has been built using 1,000 of these cells for the power unit. The Electric
Storage Battery Co. is developing a cell originated by E. Yeager at Western Reserve
University. The fuel is sodium, dissolved in mercury, which reacts with oxygen and
water to form sodium hydroxide. It will not produce cheap electricity because of
the high cost of sodium, but it may have important defense applications such as
powering a quiet submarine.
It does not require a vivid imagination to picture the possible applications
of fuel cells. Applications range from electric cigarette lighters fueled with methyl
alcohol, to heavy power production for electrical furnaces used by such industrial
giants as the aluminum, glass and steel industries. A fuel cell "burning" gasoline
or light hydrocarbons could be the power unit of an automobile if it had a satisfactory
power to volume ratio, long life and rapid startup. An electric automobile requires
no transmission: it could have electrical braking, four-wheel drive and differential
wheel speeds for safe cornering. Troubleshooting on such an automobile would be
done mainly with a test meter.
There is no doubt that the use of fuel cells will need specially designed electrical
and electronic control and testing gear plus engineers capable of using them. However,
I should like to emphasize that I have not discussed the many disadvantages and
problems remaining to be overcome in the development of fuel cells. It is possible
that many of the potentialities of fuel cells will never be reached, but fuel cells
in some form for some applications are on the way.
Further Reading
G. J. Young, Fuel Cells, Reinhold Publishing Corp., 1960.
B. R. Stein, Status Report on Fuel Cells.
ARO Report No. 1. Office of the Chief of Research and Development, Department
of Army, Washington 25, D. C. ($1.25)
Posted May 15, 2023
|