August 1964 Electronics World
Table
of Contents
Wax nostalgic about and learn from the history of early electronics. See articles
from
Electronics World, published May 1959
- December 1971. All copyrights hereby acknowledged.
|
It was not until 1963 that
the International Committee of Weights and Measures (CIPM) adopted the cesium clock
as the world scientific community's standard time reference. It boasted an accuracy
that kept it within 1.1 parts in 100 billion, meaning it would not gain or lose
more than a second in 3 thousand years. To show how far technology has advanced
since 1963, in April of 2014 the National Institute of Standards and Technology
(NIST) launched a new atomic clock called
NIST-F2 (also cesium-based) to serve as a new U.S. civilian time
and frequency standard. NIST-F2 would neither gain nor lose one second in about
300 million years - a factor of 10 thousand.
According to the U.S. Navy's official Time.Gov website, the Internet time reported on my computer was
1 minute and 37 seconds behind official U.S. time. This is not surprising since
by default Windows only re-syncs with the network once every 7 days. That means my computer
clock isn't much more accurate than a cheap old spring-driven wind-up watch. Going
into the clock settings window and clicking on the "Update" button brought the computer
clock back into sync with the NIST server clock.
Frequency & Time Standards
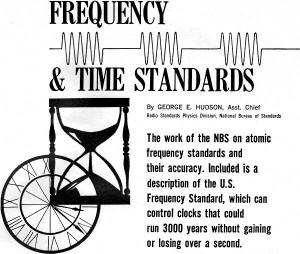
The work of the NBS on atomic frequency standards and their
accuracy. Included is a description of the U.S. Frequency Standard, which can control
clocks that could run 3000 years without gaining or losing over a second.
By George E. Hudson, Asst. Chief
Radio Standards Physics Division, National Bureau of Standards
In recent years, the National Bureau of Standards has been conducting developmental
research on the precise measurement and generation of frequency and time. For radio
communications, the tracking of satellites, the control of long-range rockets, and
astronomical observations, timing accuracies of one part in a billion or better
will be required in the future.
Many scientific and engineering activities rely on regular radio transmissions
of standard frequencies from Bureau stations WWV, WWVB, WWVL, and WWVH and from
the Navy's NBA to provide high accuracies. These broadcasts are based, in part,
on astronomical observations related to the earth's rotation as made by the U. S.
Naval Observatory. However, to meet the ever-increasing need for even greater accuracy,
the Bureau has been investigating atomic frequency standards, which are a thousand
times more precise for time-interval determinations than the earth's rotation.
A Frequency-Time Standard
Let us first consider a device which actually realizes the limits of accuracy
with which we are able to generate and determine frequencies - and times-for the
present. This is the United States Frequency Standard, illustrated on the cover
and in the various accompanying pictures and diagrams. It is located in Boulder,
Colorado, in the Radio Standards Laboratory of the Institute for Basic Standards
- one of the four Institutes which now comprise the National Bureau of Standards.
For some time now the primary standard for frequency in the United States has
been an atomic one. Up until now the most precise such standard has used a cesium
beam, undergoing a hyperfine dipole structure transition using magnetic resonance
as its controlling element. In effect, the cesium atoms are constantly spinning
in such a way as to produce magnetic poles having a certain orientation. Under certain
conditions of excitation, the atoms appear to suddenly "flip over" or reverse their
spin direction and the magnetic poles reverse.
The cesium beam frequency standard is essentially an atomic beam spectrometer
which emits a signal only when the frequency of radiation introduced through a waveguide
into the cavity through which the beam is passing is precisely equal to the resonance
transition frequency. This frequency is actually in the microwave region. The presence
or absence of the signal indicates whether the frequency of the radiation, itself
generated by a quartz-crystal oscillator driving a frequency multiplier chain, is
within the allowable tolerance limits.
In practice, the quartz signal generator is manually or automatically varied
over a narrow band to find the "center" frequency. When the spectrometer output
is at a peak, the signal generator frequency is known within ±0.1 cps or 1.1 parts
in 1011 (a hundred billion). Automatic equipment is used to control the
signal generator so that the spectrometer output stays at the maximum. This provides
a signal of known and nearly constant frequency for as long as the device can be
kept running. As the separations of the quantum states of an isolated atom are fundamental
constants of nature, they provide a stable, reproducible standard of frequency and
time interval when the atomic beam resonance technique is used.
Previously, the most uniform time-intervals available were those derived from
astronomical observations of the rotation of the earth relative to the fixed stars.
This was corrected to the orbital motion of the earth about the sun. The orbital
motion of the earth at 12 hours (noon, E.T.) on January 0, 1900 (December 31, 1899)
is the basis of what is called Ephemeris Time (E.T.). In 1956, the second of Ephemeris
Time was adopted as the fundamental unit of time by the International Committee
of Weights and Measures (CIPM) and this action was confirmed by the General Conference
on Weights and Measures in 1960.
Steps were taken by the Conference toward the adoption of an atomic standard
for time-interval. In early December of 1963, the Consultative Committee for the
Definition of the Second of the CIPM met in Paris. This committee recommended, among
other things, that the cesium frequency should serve as an international provisional
standard on which the atomic second of time should be based. Research, of course,
should continue as to the best atomic standard, but it recommended that the astronomical
second be dropped except as needed for celestial mechanics.
A time scale approximating Ephemeris Time can be made immediately available by
the use of atomic standards, quartz-crystal oscillators, and counters. In terms
of the ephemeris second, the frequency of the cesium transition has been experimentally
determined to be 9,192,631,770±20 cps. The probable error, ±20 cps (or 2 parts in
109), results from the limitations on the precision of the astronomical
measurements. Consequently, it is common practice now, and in line with the policy
that the atomic frequency transition studied at the Bureau of Standards is the primary
one for the United States, to take the frequency of the transition to be exactly
at 9,192,631,770 cps (or 9192.63177 megacycles) .
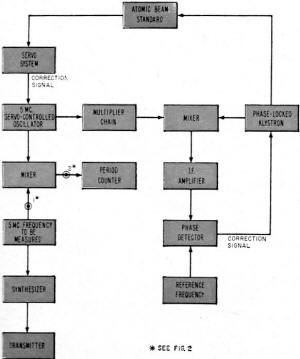
Fig. 1 - Simplified block diagram of the frequency measuring
system which utilizes the cesium beam U.S. Frequency Standard.
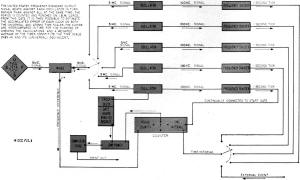
Fig. 2 - A simplified block diagram of the NBS-A atomic
time-scale generator. The U.S. Frequency Standard, shown in Fig. 1, beats against
each of the five oscillators in turn. After a time intercomparison, a weighted average
is used.
To avoid inconsistency, we must regard the error quoted of 2 parts in 109
to be the error in determination of the ephemeris second in terms of an exactly
defined atomic second. Such a definition is being currently considered. If the cesium
transition is adopted for this purpose, one second of atomic time will consist of
9,192,631,770 cycles of the electromagnetic radiation absorbed or emitted by a cesium
atom in changing its state. The physical picture in this particular case is that
the magnetic dipole moment of the cesium atom changes because of a spin transition.
Measurement of a frequency or a time-interval in terms of the cesium transition
can be made with a typical precision of 1 part in 1012 and is not limited
by the instrumental difficulties involved in astronomical observations. See Fig.
1. Until official action is forthcoming it can not, however, supplant the present
definition of a scale for time based on the non-uniform apparent motion of the sun.
It seems natural to base the standard of time-interval on the physical process
that provides the most uniform and most accessible interval. The precision of measurement
for the atomic standards is a hundred times better over a 15-second period than
astronomical measurements made over a period of 3 years.
Also under investigation as a standard of frequency is the thallium atom, which
has certain significant advantages over the cesium atom in this application. However,
thallium may have some disadvantages in practical use. A thallium beam is now in
operation at the Boulder Laboratories to determine which of the two atomic systems
is more suitable.
Radio Transmissions
At the present time, radio transmissions controlled by the Bureau's master quartz-crystal
oscillators are being monitored with cesium beam frequency standards. Corrections
for the 60 and 20 kc. standard frequency broadcasts from NBS radio station VVWVB
and WWVL at Boulder, Colorado, are being made regularly and are available on request
from the Broadcast Service Section of the Bureau's Boulder Labs.
The time generation system now employed at the Boulder Laboratories comprises
the most accurate clock that man has ever known. Fig. 2 is a schematic diagram
of this system, which generates the NBS-A time scale. W. Atkinson, L. Fey, and J.
Barnes have been chiefly responsible for its development.
Recent comparisons with other time scales - such as those of Essen in England
and particularly Bononomi in Switzerland - the so-called TA1 time scale
- have shown agreement to within 1 part in 1011 over about a two-year
period. This means that these time scales diverge at the rate of 1 second in 3000
years.
One of the time scales that is computed at the Naval Observatory from astronomical
observations is designated as UT-2. It is an approximation to Ephemeris Time and
represents an average scale obtained by correcting the earth's
period of rotation on its axis. After correcting for longitude effects on the
astronomical observations which yield UT-0 directly, one obtains UT-1. Then correcting
for seasonal variations in rotation speed one obtains UT-2. The U. S. Naval Observatory
obtains a composite "atomic" time scale - known as A1, by taking a weighted average
of various atomic standards from the United States and European laboratories. The
average is obtained from broadcast transmissions. However, propagation effects lead
to many technical questions which remain to be investigated: The Naval Observatory
publishes the difference between A1 and UT-2 regularly.
On the basis of the NBS-A atomic scale which has been kept continuously since
the Fall of 1957, it is known that the UT-2 scale, which really is simply an average
representation of the earth's rotation, shows that the earth has slowed down by
about 2.9 seconds in this time. It is clear that the uniform atomic time scale NBS-A
generated at the Boulder Laboratories and based on the atomic frequency standard
must be heavily relied on for the serious business of accurate time-keeping. It
is also clear that the United States is maintaining its eminence in this field.
Principles of Time Keeping
The principle of keeping time with an atomic clock is simple. If one has a continuous
record of the frequency, f, of any oscillator and a concurrent record of the number
of cycles of vibration, or phase of the oscillator, then the time interval between
two given phases can be calculated. If one plots the quantity 1/2πf versus the
phase, the time interval is just the area underneath the curve between the two phase
values.
Now to do this one must have a continuously running oscillator, or several oscillators
(for redundancy and to increase statistical reliability) and measure their frequencies
periodically by comparison with the atomic standard. Then one must also count the
cycles generated by the oscillators with a suitable counting circuit. The NBS-A
time scale is generated essentially in just this way. To account for the tiny variations
in frequency of the oscillators used (four quartz ones and one rubidium one) intensive
studies have been and are being made of their physical and their statistical characteristics.
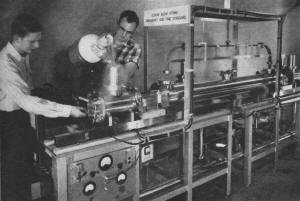
Scientists are shown above pouring liquid nitrogen
(at a temperature of -320° F) from a Dewar into
one of the cold traps in the NBS "atomic clock" at the Boulder Laboratories. The
nitrogen lowers the temperature in the evacuated tube and causes stray gas molecules
and other impurities to condense around the cold end of the trap. This reduces the
chance of collision between the cesium atoms and molecules of air, thus improving
the vacuum and increasing cesium-beam accuracy.
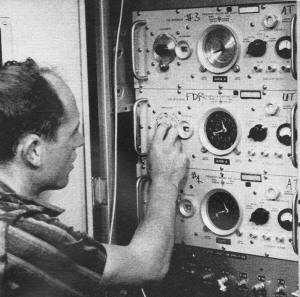
An NBS scientist is shown retarding by 0.1 second on April 1,
1964 the clock which maintains Universal Time (UT) at the Boulder Laboratories.
This 100-millisecond adjustment was necessary due to changes in speed of rotation
of the earth, as determined by astronomical observation. Center clock (being adjusted)
is kept in close agreement with UT-2 (GMT). The top and bottom clocks, which are
controlled by the U.S. Frequency Standard and which are not adjusted, maintain atomic
time and were in agreement with UT-2 on Jan. 1, 1958, as determined by the U.S.
Naval Observatory. Since that time, the clock maintaining UT has lost about 2.9
seconds relative to atomic time. Since UT-2 is determined by the earth's rotation
(which is slowing down), the center clock must be retarded periodically and progressively
loses time (150 x 10-10 sec./sec. for 1964).
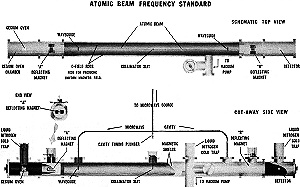
Atomic Beam Frequency Standard (see magazine
cover photo)
The result is a clock whose accuracy in keeping time is only limited by the accuracy
of the U.S. Frequency Standard itself. There is not one but five complete time keeping
systems in the fail-safe setup shown in Fig. 2. A weighted average of the five
leads to the NBS-A atomic time scale.
Signals from this system control the broadcast frequencies and time furnished
by the Bureau, under the direction of Mr. D. Andrews. A recent innovation utilizing
a phase-lock system insures that the time signals emitted by WWV are in phase with
the NBS-A scale to within 25 microseconds. It is only at certain intervals, as the
earth gradually changes its rate of rotation, that shifts must be broadcast so that
all the signals simultaneously are kept in agreement with UT-2 furnished by the
Naval Observatory. Between times, the rate of time ticks is atomically controlled.
Thus the Bureau of Standards must maintain two time scales simultaneously under
its NBS-A system.
Atomic Beam Spectrometer
The atomic beam spectrometer, which is the heart of our frequency and time measuring
system, is shown in cross-section in one of the photos. Neutral cesium atoms effuse
from the oven and pass through the non-uniform magnetic field of the deflecting
magnet. As the atoms act as miniature bar magnets (they are said to have a magnetic
dipole moment), a transverse force will act upon them in this non-uniform field.
The magnitude and direction of this force depends upon which of the energy states
the particular atom is in. Of all the atoms effusing from the oven, suppose those
with nuclear and electron spins in the same direction, say up, have their trajectories
bent toward the axis. Atoms on the other side of the center line with spins in opposite
directions, with the net being down, will have their trajectories bent toward the
axis also. Note that atoms with upward spins and those with downward spins experience
forces in opposite directions. The upward-spin atoms and the downward-spin atoms
will cross the axis at the collimator slit, pass through the slit, and enter the
region of the B deflecting magnet.
AAs the B magnetic field is exactly like that of the A magnet, it exerts the
same transverse force on the atoms as does the A magnet field. The upward-spin atoms
will experience a downward force as before and the downward-spin atoms will be
acted on by an upward force, as before. As the two sets of atoms are now on opposite
sides of the center line of the evacuated tube (compared to their previous positions),
these forces make their trajectories more divergent.
However, if a radiation field is applied at just the proper frequency (which
matches the energy separation of the two quantum states), transitions between the
two states will occur in the region between the A and B magnets. The spins will
be reversed in the two beams, and a quantum of energy will be either emitted or
absorbed. Since the sign of the magnetic moment has changed in moving from the A
magnet to the B magnet, the force on these atoms will reverse its direction and
the atoms will be re-focused onto the axis at the detector. Thus, as the exciting
radiation is swept in frequency, the detected signal will increase and reach a maximum
at the critical frequency, and then decrease as the radiation frequency is varied
on either side.
We have tried to furnish some notion of the intense activity which is now current
at the Boulder Radio Standards Laboratory of the National Bureau of Standards' Institute
of Basic Standards. Highly accurate atomic standards exist at the Bureau-but they
are constantly being improved and modified as the state of the art progresses.
A hydrogen maser is under development and will soon be ready for comparison with
the cesium beam frequency standard. Commercial varieties are now available which
hold considerable promise of being at least competitive with the presently used
standard.
The adoption of the definition of the atomic second as the international standard
of time seems to be in the immediate offing. The NBS atomic frequency standard
itself controls the rate of the very accurate atomic time scale and the broadcast
information, whereby industry and the scientific community can feel confident that
it is receiving the best and most up-to-date services available in the area of frequency
and time.
The author wishes to express his thanks for and acknowledgement of the contributions
made in the writing of this article to his associates at NBS (BL).
Cut-away scale drawing of the NBS cesium beam frequency standard, or "atomic
clock," shows the location of the cesium oven at the left end where the cesium atoms
are emitted; the deflecting magnets at each end which bend the beam and direct it
through the collimator slit to the detector; the microwave cavity and waveguide,
which introduce the microwave frequency; the three liquid nitrogen cold traps used
to remove, by condensation, impurities not removed by the vacuum pump; the magnetic
shields, designed to eliminate the influence of terrestrial and other outs ide magnetic
fields; the hot-wire detector at far right end where the beam impacts and is detected.
This equipment is essentially an atomic-beam spectrometer excited by a crystal oscillator
driving a frequency-multiplier chain. The radio waves, oscillating a t a frequency
close to the vibration-per-second rate of the cesium atoms (9192.63177 me.I, are
tuned slightly so that they match the atomic rate. When they match, the radio waves
are absorbed; the radio frequency is then the same as that of the cesium atoms-and
a near absolute standard is available. The device can measure an unknown frequency
with an accuracy of 1.1 parts in 1011 (one hundred billion). This is
equivalent to measuring the width of the United States with less error than the
thickness of this page. Its stability is even higher-at least a few parts in 1012
per year-and at the present time these figures represent one of the best achievements
in this field in the entire world.
Posted October 4, 2023 (updated from original
post on 3/4/2015)
|